EVOLVING CRATONS
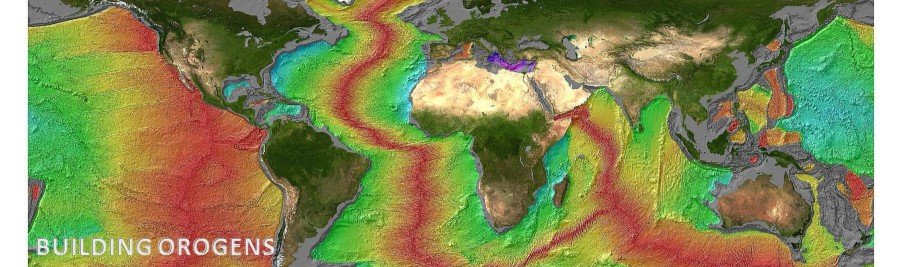
The South American and African continents are riddled with a number of continental blocks (or cratons) that have been shielded from mantle convection by thick roots of mantle lithosphere.
A common feature to all these cratons is that they preserve Archaean crustal segments (or shields) that were formed between 3.5 and 2.5 billion years ago. These ancient shields assembled into broad, continental-scale cratons via tectonic accretion and subsequent partial melting in the Palaeo- and Mesoproterozoic. However, little is known about the timing and the processes that converted the shields into solid cratonic units since the Archaean.
The main research activities are:
- Constrain magmatic/metamorphic ages of Archaean granitoids/gneisses and associated volcanic rocks within the shields
- Geochemical modeling of Archaean gneisses and granitoids that form the bulk of the shields
- Detrital zircon age dating of greenstone belt sequences
- Construct the metamorphic evolution of greenstone belt pelites and amphibolites via P-T-t modeling – Theriak Domino and Thermocalc
- Link peaks of granitoids magmatism and high-grade metamorphic events within these cratonic shields
Craton stabilization and magmatism
The withdrawal of large volumes of potassic (K-rich) magmas from the deep continental crust and their emplacement at higher structural levels is a common process observed in many Archaean provinces worldwide. This type of magmatism generates high-K, calc-alkaline granites and granodiorites, which often occur later in the tectonic history of the provinces, following the assembly of older tonalite-trondhjemite-graniodiorite (TTG) crust. The majority of these granitoids are broadly coeval with or post-date compressional deformation and crustal thickening, and are commonly linked to either crustal thickening and anatexis (during late stages of collision of TTG crust) or decompressional melting due to upper-crustal extensional collapse of thickened orogens. Irrespective of the tectonic process, the massive production of such granitoids has a substantial effect on the stability of the lithosphere because it reduces the long-term heat production in the deep crust by removing heat-producing elements (HPEs) from the deep crust. Partial melting and upward migration of felsic magma also generates a strong rheological column within the crust and strengthens the upper crust by crosscutting pre-existing tectonic weaknesses. Consequently, voluminous potassic magmatism should lead to the formation of cold and rigid continental blocks that are able to sustain the deposition of large sedimentary covers.

Fig 2 – Simplified geological map of the Sao Francisco Craton
In the southern tip of the São Francisco Craton (Fig. 2), southeastern Brazil, potassic granitoids together with tonalite-trondhjemite-granodiorite (TTG) rocks and greenstone belt strata comprise one of the oldest Archaean continental shield areas of South America. The potassic granitoids are demonstrably younger than the TTG counterparts and their widespread distribution seems to mark a distinct change in the tectonothermal regime of the crust during the growth of the craton in the late-Archaean. A significant portion of the potassic granitoids remained undeformed since crystallization; they testify to the main crustal thickening event during stabilization of the Archaean crust.
This research is focussed on three main issues:
- the ages and nature of the source(s) undergoing melting
- the processes that have shaped the geochemistry of the magmas
- the processes which lead to heating and melting of the lower crust
The Quadrilátero Ferrífero
The Quadrilátero Ferrífero (QF) province, in the Southern São Francisco Craton (SSFC), exposes a large segment of Mid- to Neoarchaean continental crust that served for many years as type locality for understanding early crustal evolution in South America. The Archaean history of this segment spans about 600 My, and can be subdivided into two fundamentally distinct stages. The first stage, lasting more than 400 My, involved the emplacement of voluminous TTG magmas and extrusion of mafic and ultramafic rocks between 3200-2770 Ma (Fig. 3). The TTG magmatism led to the construction of a regionally extensive TTG crust riddled with numerous greenstone belt occurrences. The second stage was marked by high-grade ductile deformation, partial melting of the TTG-greenstone crust, and concomitant emplacement of voluminous potassic granitoids between 2750-2600 Ma. This period of intra-crustal melting saw the emergence of a stable continental platform that was substantially rigid to sustain the deposition of thick Palaeoproterozoic sequences, including the extensive banded iron deposits of the Quadrilátero Ferrífero mining district (Fig. 3).
Recent work indicates that this sort of model where the K-granites are derived from TTG anatexis is very likely to be incorrect. TTGs are quite low in heat-producing elements, they also are not the source of the granites. Formation of the granites requires either K-rich mantle melts interacting with the lower crust during anatexis, maybe a combined heat source and major flux of mantle-derived material into the crust, or burial of K-rich sediments by an orogenic process. For BGB I favor the latter. Rocks like the volcaniclastic felsic units of the TheespruitFm and the Fig Tree group are the source of the 3.14 to 3.10 granites in BGB.
Fig. 3 – Schematic evolution of the Southern São Francisco Craton in the Late-Archaean. a) Undifferentiated crust recording concomitant deposition of the Rio das Velhas Group Lavas and emplacement of the younger TTG suites at ca. 2780-2770 Ma. b) Emplacement of potassic granitoids at 2760-2700 Ma. This process led to differentiation of the crust into a refractory lower crust and an upper crust enriched in heat-producing elements HPE. c ) Subsequent redistribution of the HEP during erosion and peneplanation of the upper crust and accumulation of the Minas Supergroup clastic sediments
Objectives
The proposed study will pursue high-precision geochemical and isotopic analyses of the potassic granitoids in order provide detailed information about compositional diversity within them, the sources of their chemical diversity, details of the time span of crystallization of the plutons and, finally, modeling of geodynamic scenarios leading to the production of high volumes of potassic magmas. We suggest the following goals within the context of the post-doctoral project:
- 1 – Characterize the geochemical diversity between the plutons through XRF and LA-ICP-MS analyses. Major and trace element analyses will be accompanied of detailed field and petrographic observations of granitoids exposed in several (8-10 would probably be sufficient) localities that cover most of the geographical range of the main batholiths in the region.
- 2- Constrain the nature of the primary source or sources of the potassic magmas via isotope studies. Analysis of the U/Pb ages and Hf-Lu isotopes will be carried out on a sufficiently large number of inherited zircons, titanites and/or monazites (~ 200) from selected samples. A similar dataset should be acquired for the TTG rocks exposed in the interior of the granitoid-gneiss complexes.
- 3-Establish the duration of the magmatic process via detailed zircon geochronology of the magmatic zircons. This will be combined with Hf-Lu isotope analysis of the magmatic zircon fraction from different areas to define how homogenous was the magmatic source of these granitoids.
- 4-Define the U-Th and other REE element content of magmatic accessory phases and xenocrystals in other to understand the partitioning of these elements in the source and the phase melt.